Even if the number of electric vehicles (EVs) on the road is rising, automakers are nevertheless aware of the obstacles preventing their widespread acceptance. Manufacturers are attempting to overcome the cost, safety, performance, and engineering difficulties related to EV batteries at the same time that the industry addresses the lack of charging infrastructure and supply chain security.
Globally automakers are planning to spend more than half a trillion dollars on EVs and batteries through 2030, according to a recent Reuters analysis. This is up from $300 billion noted by the same study conducted three years earlier.
High on the list of challenges are well-documented consumer concerns regarding limited range, time-to-charge, cost, safety, and lifespan – all primarily related to limitations of current lithium-ion battery (LIB) technology. To overcome these limitations automakers are increasingly viewing battery physics and architecture as the next big battery technology breakthrough.
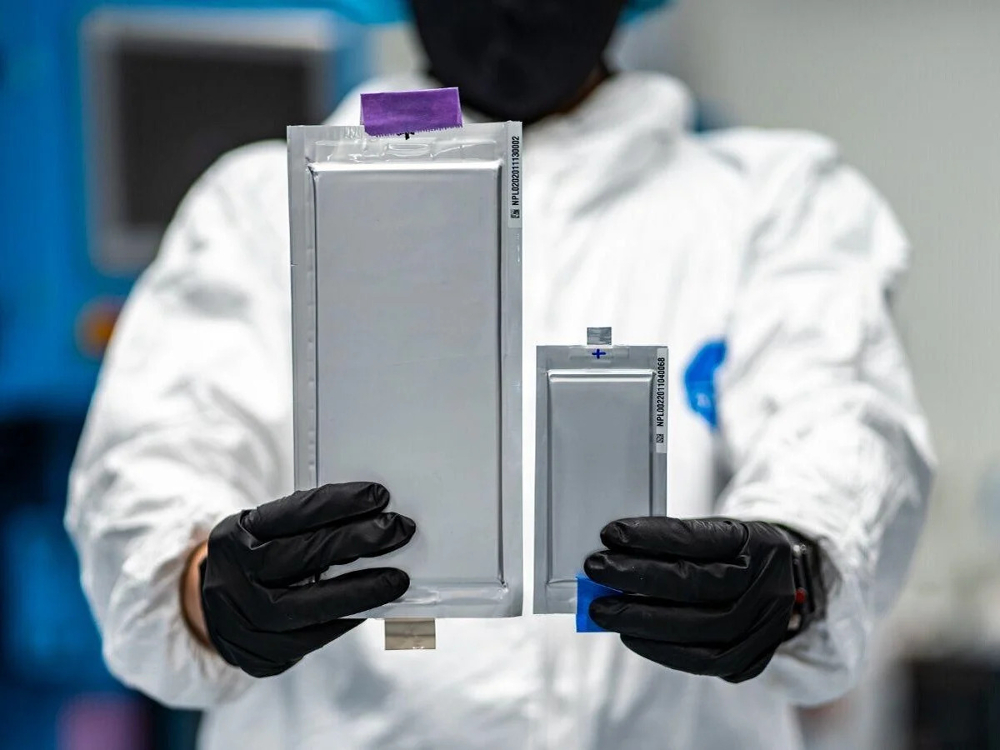
A solid-state battery is a battery technology that uses solid electrodes and a solid electrolyte, instead of the liquid or polymer gel electrolytes found in lithium-ion or lithium polymer batteries. The industry opinion has long been that all-solid-state batteries have the prospect to increase energy density and reduce the time to charge, while improving safety - in particular run-away thermal events. This is largely due to the adoption of a solid electrolyte (SE) that would lessen the flammability associated with current solvent-based liquid and gel electrolytes.
This is of particular interest when considering the use of lithium metal as an anode, that when paired with a sulfur cathode, could potentially deliver an energy density 2 to 3 times greater than batteries currently available.
Even though lithium-metal anodes hold great promise to improve energy density, liquid or gel electrolyte batteries with these electrodes suffer from poor cycle life and carry a higher risk of dendrite formation and short-circuits.
Lithium dendrites are metallic microstructures that form on the negative electrode during the charging process and they are formed when extra lithium ions accumulate on the anode surface and cannot be absorbed into the anode over time.
Therefore the overall risk of using lithium-metal instead of a silicon based anode for example, might be too high for the electric vehicle. The Lithium metal itself is highly reactive, and combining it with a potential failure of the car or an unfortunate impact as a result of an accident makes this solution highly questionable. Alternatively, some companies have opted to remain with the silicon anode as the negative side of the battery, and to continue to use the Lithium ions in the non-metal state.
The switch to a solid electrolyte in an all-solid-state battery is seen as a possible solution to overcome these problems.
Challenging the perception of the superiority of all-solid-state and solid-state batteries in EV applications
In all-solid-state batteries, both the electrodes and the electrolytes - which can be composed of any number of everyday materials, like ceramics and glass - are solid-state, with the SE serving as a separator as well. The inherent safety of a non-volatile SE gives all-solid-state batteries the potential to be safer, have greater energy density – thereby enabling a weight reduction - and offer more range.

Enhanced safety also means less safety monitoring electronics are needed in the battery packs, while increased stability could allow for faster charging compared to liquid or gel lithium-ion batteries
The replacement of liquid electrolytes with SEs can also serve as another avenue to realize safer, long-lasting batteries as they are more resistant to physical changes under varying temperatures.
Nevertheless, all-solid-state batteries face several performance and manufacturing obstacles.
For instance: A key consideration in manufacturing a battery is the packing density of the cathode, which is critical to reaching high energy density and low cost. However, in the all-solid-state battery, mixing SE with cathode particles requires high pressure and temperature, while keeping the process moisture-free, resulting in higher production costs. In addition, standard SEs are often brittle experiencing ductile failure during manufacturing or use in EVs. This characteristic requires the cells to be kept under constant pressure during operation.
Whilst the SE in the all-solid-state battery may hold the promise of a safer fast charging battery, because of the absence of the more flammable liquid or gel electrolyte, its ionic conductivity is lower, severely curtailing the fast charging and discharging potential. The Li-metal anode further aggravates the problem with the Li-ion diffusion in the anode being slow.
For this reason, all-solid-state battery researchers are studying ways of adding small amounts (<8%) of liquid electrolytes. However, by adding liquid electrolyte the safety advantage of the all-solid-state battery is reduced in what is commonly referred to as the solid-state-battery or semi-solid-battery (SSB).
Moreover, intensive research on the safety of all-solid-state batteries has raised a question about whether EVs with SEs can guarantee absolute safety. Thus, a recent study led by the U.S. DOE Sandia National Laboratories, published in the scientific journal Joule, found that the solid electrolyte can still fail under certain circumstances, such as when the battery is crushed, punctured, or when built-up pressure causes a reaction between internal oxygen and lithium.
So, with limited independent verification of the advantages of solid-electrolytes in all-solid-state batteries Stan Whittingham, 2019 Nobel Laureate in Chemistry, opened the 2022 Materials Research Society's Spring Meeting with a presentation on “The Pros and Cons of Solid vs. Liquid Electrolytes in Lithium Batteries”. He reframed several statements about SEs in solid-state batteries, which many have taken as foregone conclusions, into open questions: Will solid electrolytes lead to higher energy density, greater safety, cleaner and more sustainable manufacturing, and less thermal sensitivity?
The latest Li-ion batteries challenge solid-state technology
Even though all-solid-state batteries theoretically offer the potential to overcome many of the current Li-ion battery's limits, the technology is yet to be fully commercialized and industrialized, with current claims mostly unverified.
Notwithstanding the use of a lithium-metal anode in the ASSB/SSB to boost energy density - which because of its high energy density and unstable nature will continue to pose a safety risk irrespective of the electrolyte, and therefore may be years away – current Li-ion batteries continue to make significant advances.
Already StoreDot's extreme fast charging (XFC) EV LIB technology, using silicon-dominant anodes, is capable of achieving an energy density of 300Wh/kg and 680 Wh/l in real-world conditions at room temperature, whilst retaining 70 percent of their original capacity after 1700 cycles.
SEs used in all-solid-state batteries are also approximately 2 to 4 times heavier than liquid electrolytes and require significant external pressure for optimum operation – necessitating specialized battery enclosures that add weight and further reduce specific energy density.
LIBs also hold a significant advantage over solid-state batteries in cost. All solid-state batteries are expected to cost $80-90/kWh by 2030, while the price of lithium batteries is expected to reach $60/kWh in the same time frame.
So, at the end of the day, all-solid-state and solid-state technologies may well offer too little in the way of charging and in the case of SSBs, safety advantages over the latest Li-ion batteries – although they may achieve a higher energy density.
StoreDot's semi-solid approach to enhancing energy density
The core focus of StoreDot in the future development of an Extreme Energy Density (XED) product is based on a SE with organic and inorganic components, utilizing an ionic conductor in the liquid or polymer phase, such as a gel.

The semi-solid approach allows the electrolyte to be optimized for application at the anode and cathode interfaces. Furthermore, semi-solid architecture naturally emerges as a potential solution to rate capability and high-temperature processing compatibility issues, without applying external pressure during cell operation. Furthermore, such a design still has advantages from a safety perspective by reducing the volume of flammable liquid electrolytes in comparison to a typical liquid system.

Summary
Even though solid-state- and all-solid-state-batteries promise cost-effective fast and safe charging batteries, with high energy densities they remain a work in progress, and, faced with many significant challenges a realistic date for a possible debut still remains uncertain. In the case of the all-solid-state battery, the challenges around the use of solid state electrolytes may well require other future solutions such as StoreDot's semi-solid battery.
The solution lies in the combination of the benefits of solid state electrolyte (such as catholyte and anolyte), while maintaining a small liquid or gel form of electrolyte that allows for a better flow of the ions between the cathode and anode, and mitigates the critical issue of solid-to-solid interface that otherwise holds too high of resistance. In the near future we will start seeing more battery solutions that are based on this semi-solid approach which essentially leverages on the best of both worlds – the semi-solid electrolyte and the silicon anode.